Reporting by Helen Hill for the MIT Darwin Project
For the past several years, Rogier Braakman, a research scientist working in Penny Chisholm’s lab and collaborating with Mick Follows, has been studying how metabolism evolves in ocean microbes. In a new paper, he argues that intrinsic properties of cellular metabolism imposed central constraints on the historical trajectories of biospheric productivity and atmospheric oxygenation.
In an earlier paper (Braakman et al 2017, reported by MIT News), Braakman pieced together the evolutionary history of the abundant marine cyanobacterium Prochlorococcus by drawing on the genes present in different ecotypes and the conditions in which they live and developing a framework for how this shaped the ocean ecosystem. In a new follow-up paper, he further examines how metabolic innovations in ancestors of these microbial cells hundreds of millions of years ago shaped the Earth system as a whole, and whether this could have contributed to the observed explosion of life outside the oceans by drawing down atmospheric carbon dioxide and increasing oxygen in the air.
In his new paper, now available online in the journal Free Radical Biology and Medicine, Braakman argues that intrinsic properties of cellular metabolism imposed central constraints on the historical trajectories of biospheric productivity and atmospheric oxygenation. “Photosynthesis depends on iron, but iron is highly insoluble under the aerobic conditions produced by oxygenic photosynthesis and these counteracting constraints led to two major stages of Earth oxygenation,” he explains. “After a cyanobacteria-driven biospheric expansion near the Archean-Proterozoic boundary, productivity remained largely restricted to continental boundaries and shallow aquatic environments, where weathering inputs made iron more accessible.”
“The anoxic deep open ocean was rich in free iron during the Proterozoic, but this iron was largely inaccessible, partly because an otherwise nutrient-poor ocean was limiting to photosynthesis, but also because a photosynthetic expansion would have quenched its own iron supply,” says Braakman. “However, near the Proterozoic-Phanerozoic boundary, bioenergetics innovations allowed eukaryotic photosynthesis to overcome these interconnected negative feedbacks and begin expanding into the deep open oceans and onto the continents, where nutrients are inherently harder to come by.”
Braakman says key insights into what drove the ecological rise of eukaryotic photosynthesis emerge from analyses of marine Synechococcus and Prochlorococcus, abundant marine picocyanobacteria whose ancestors colonized the oceans in the Neoproterozoic. His work reconstructing the evolution of this group reveals a sequence of innovations that ultimately produced a form of photosynthesis in Prochlorococcus that is more like that of green plant cells than other cyanobacteria.
Braakman explains, “Innovations increased the energy flux of cells, thereby enhancing their ability to acquire sparse nutrients, and as a by-product also increased the production of organic carbon waste. Some of these organic waste products, in turn, had the ability to chelate iron and make it bioavailable, thereby indirectly pushing the oceans through a transition from an anoxic state rich in free iron to an oxygenated state with organic carbon-bound iron.” Resulting conditions (and parallel processes on the continents) further led to a series of positive feedbacks that increased the availability of other nutrients, thereby promoting the rise of a globally productive biosphere.
In addition to the occurrence of major biospheric expansions, Braakman notes, the several hundred-million-year periods around the Archean-Proterozoic and Proterozoic-Phanerozoic boundaries share a number of other parallels. For example, both epochs have also been linked to major carbon cycle perturbations and global glaciations, as well as changes in the nature of plate tectonics and increases in continental exposure and weathering.
Braakman interprets this as evidence that the dynamics of life and Earth are intimately intertwined across many levels and that the general principles he outlines governed transitions in these couples dynamics at both times in Earth history.
To find out more about this work contact Rogier
Publication
Rogier Braakman (2019), Evolution of cellular metabolism and the rise of a globally productive biosphere, Free Radical Biology and Medicine, doi: 10.1016/j.freeradbiomed.2019.05.004
Related
Tiny Bacterium Provides Window into Whole Ecosystems MIT News
Video: “Meet Prochlorococcus” Science Magazine
About the Researcher
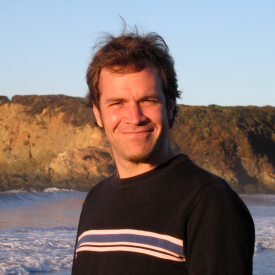
Rogier Braakman is a Research Scientist in the Chisholm Lab and has, for many years, collaborated with Mick Follows as part of the MIT Darwin Project. He holds a Master’s degree in chemistry from the University of Amsterdam and a PhD in chemical physics from the California Institute of Technology. Braakman’s first postdoc was at the Santa Fe Institute, where he began studying metabolism, subsequently moving to MIT to learn more about microbial ecology and evolution and Earth history.
“I have always been interested in the evolution and self-organization of chemical systems in nature and have worked on this in a variety of contexts,” says Braakman. “As an undergraduate, I read a lot about evolution and took various classes in history and astronomy, all around the goal of wanting to place my thinking about chemical systems (and complex systems more generally) in a more evolutionary/historical context. Eventually, this led to me pursuing a PhD in the field of ‘astrochemistry’, where I focused on studying the organic chemical processes that occur in star-forming regions in the interstellar medium. I wanted to understand how chemical complexity arises in these environments in general, and how, in the case of our solar system, this may have seeded the early Earth with the precursors to life.”
By the end of his PhD Braakman realized that the gap between prebiotic chemistry and life was too wide for him to satisfactorily bridge so he decided he might be better off studying it directly. This led him in the direction of biology at the Santa Fe Institute, a theoretically-focused institution perhaps best described as a kind of interdisciplinary science “think tank”. There, surrounded (and influenced) by archeologists, economists, computer scientist and biological theorists looking for general principles of complex systems, Braakman says he began studying metabolism. “Initially I wanted to trace metabolism back to its roots on the early Earth to understand connections to the prebiotic chemistry I had studied previously,” he says. “But, the more I studied metabolism the more I began to see connections to ecology, and I realized that I didn’t know how to think like an ecologist, or more generally how to think about the emergence of higher levels of organization in the biosphere. Ultimately this motivated my move to MIT to be part of the broader ecology community around Penny and Mick’s groups.”
Since coming to MIT, in addition to finding a great system (oceanic microbial ecosystems!) to study the questions that interest him, Braakman’s goals further broadened, from studying ecology to also studying oceanography, geochemistry, and Earth history. “This was all driven by the realization that to study life you really needed to think about what is happening across multiple scales of space and time and biological organization, says Braakman. “It might make-for somewhat ‘slow’ science, but I find it deeply gratifying.”